Flooding occurs largely based on clear and quantifiable municipal infrastructure design standards that have evolved over the last century, steadily reducing flood risk. Certainly factors such as urbanization and intensification affect hydrology and increase runoff and risks, even when rainfall intensities have not changed (e.g., southern Ontario for example) - but fundamental design characteristics that may or may not account for extreme weather effects are the predominant factor affecting urban flood risk.
From Flood Plains to Floor Drains, my unifying theory of urban flood risk, described how planning and design practices have evolved within the realms of riverine flooding (i.e., flood plain management), pluvial flooding (i.e., major overland drainage design), and sewer back-ups (i.e., wastewater and stormwater sewer design). These systems may also interact during extreme weather through processes not explicitly considered in the design and that may accentuate core design limitations, or lower levels or service, in the related system. For example, flood plains may back up into sewer systems (e.g., Carp River, Ottawa or Etobicoke Creek, Toronto). Or overland drainage systems may overwhelm sanitary sewer systems with inflows ... insert your local 'Lost River' example here.
So what are the construction eras and infrastructure servicing standards that characterize extreme weather flood resiliency or risk? Here is an approximate grouping that I developed to support a white paper on Core Public Infrastructure knowledge gaps and research needs for the National Research Council last year. That work did not look into riverine systems but those are included here:
Servicing Era 1 - 1960 and before
Median Flood Risk =
HIGH (4 out of 5)
Level of Service Profile:
- Riverine flood risks are not uncommon, unless structural controls have been put in place (e.g., dams, berms, etc) or land use planning has relocated original at-risk dwellings.
- Pluvial / overland flood risks exist as it was not a common design practice to accommodate major system flows during extreme rainfall events.
- Sewer back-up risks exist due to high extraneous flow stresses during extreme rainfall events in combined wastewater systems and partially-separated systems (i.e., with foundation drains / weeping tiles connected to the sewer system). Risks may increase if there is reliance on mechanical and electrical systems (e.g., pumping stations in the collection system) or may decrease if hydraulic relief is available through combined sewer overflows (CSOs) or storm sewer overflows (SSOs).
Other Considerations:
- CSO or SSO hydraulic relief may have a relatively-smaller moderate flood risk (3 out of 5)
- Systems serviced by pumping systems that have finite capacity or that are affected by flood plain encroachment may have a relatively-higher highest risk (5 out of 5).
Servicing Era 2 - 1960 to 1980
Level of Service Profile:
- Riverine flood risks vary overall according to natural hazards land use planning (provincial or local policies) and vary locally based on the spatial extent of flood risk mapping (i.e., have large drainage areas (up to about 125 hectares) been mapped or estimated including through urban areas.
- Pluvial / overland flood risks exist as it was not a common design practice to accommodate major system flows during extreme rainfall events.
- Sewer back-up risks exist due to high extraneous flow stresses during extreme rainfall events in partially-separated systems (i.e., with foundation drains / weeping tiles connected to the sewer system). Risks may increase if there is reliance on mechanical and electrical systems (e.g., pumping stations in the collection system).
Median Flood Risk =
HIGHEST (5 out of 5)
Other Considerations:
- Systems with good overland catchment slopes may have a relatively-lower high flood risk, despite no explicit overland drainage system (4 out of 5).
- Systems serviced by pumping systems that have finite capacity or that are affected by flood plain encroachment may have a relatively-higher risk.
Servicing Era 3 - 1981 to 1990
Level of Service Profile:
- Riverine flood risks may be significantly reduced according to natural hazards land use planning (provincial or local policies) and can vary locally based on the spatial extent of flood risk mapping (i.e., have large drainage areas (up to about 125 hectares) been mapped or estimated including through urban areas.
- Pluvial / overland flood risks may exist from jurisdiction to jurisdiction as this design practice was introduced to accommodate major system flows during extreme rainfall events, often in combination with master drainage planning at the early land use planning stage.
- Sewer back-up risks are limited due to low extraneous flow stresses during extreme rainfall events in fully-separated systems (i.e., no foundation drains / weeping tiles connected to the sewer system). Risks may increase if there is reliance on mechanical and electrical systems (e.g., pumping stations in the collection system).
Median Flood Risk =
MODERATE (3 out of 5)
Other Considerations:
- Systems with good overland catchment slopes, often through explicit dual-drainage design for major system design, may have a relatively-lower low flood risk (2 out of 5).
- Systems serviced by pumping systems that have finite capacity or that are affected by flood plain encroachment may have a relatively-higher highest risk (4 out of 5).
Servicing Era 4 - 1990 to today
Median Flood Risk = LOW (2 out of 5)
Level of Service Profile:
- Riverine flood risks are typically significantly reduced according to natural hazards land use planning (provincial or local policies) . Local and downstream risks may be reduced through integrated land use and watershed/subwatershed planning.
- Pluvial / overland flood risks are limited where the dual-drainage design practice was introduced to accommodate major system flows during extreme rainfall events, often in combination with master environmental servicing (including drainage) planning at the early land use planning stage.
- Sewer back-up risks are limited due to low extraneous flow stresses during extreme rainfall events in fully-separated systems (i.e., no foundation drains / weeping tiles connected to the sewer system). Risks may increase if there is reliance on mechanical and electrical systems (e.g., pumping stations in the collection system).
Median Flood Risk =
MODERATE (3 out of 5)
Other Considerations:
- Systems with no explicit dual-drainage design for major system design may have a relatively-higher high flood risk (4 out of 5).
- Systems serviced by pumping systems that have finite capacity or that are affected by flood plain encroachment may have a relatively-higher highest risk (4 out of 5).
Servicing Era 4 Plus - Added Enhanced Best Practices to Servicing Era 4
Median Flood Risk = LOWEST (1 out of 5)
OK, so what are the enhanced best practices that create Era 4+ ? Basically take the good practices in Era 4 and add measures that provide enhanced resiliency in each of the realms.
Enhancements:
- Riverine flood risk reduced through adoption of higher return period events (e.g., above 100-year level of service) or significant freeboard allowances (safety factors in design).
- Pluvial / overland risk reduced through provision of adequate freeboard on major drainage system to prevent entry to properties.
- Sewer back-up risks reduced through mandatory plumbing system isolation (backwater valves and sump pumps), or robust hydraulic design of gravity systems to consider extreme rainfall stresses above 100-year level (e.g., including future projected rainfall intensities) and to consider freeboard to basement systems during extreme events, and inlet control devices to limit storm sewer system surcharge.
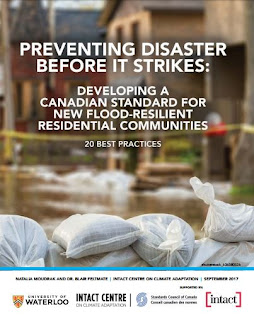
A longer list of enhanced-level best practices is found in the Intact Centre on Climate Adaptation seed document on Best Practices for New communities:
So how do we know these Servicing Eras are relevant and really do affect flood risk? By using data to track reported flooding and correlating the flood density to the servicing era or the characteristic within the servicing era. An example of this is my assessment of overland flow characteristics, and catchment slope characteristics on reported Toronto flood density:
And reviews of flood density in Toronto based on era of construction (I used watermain installation date as a surrogate) (see slide 36):
Or in Markham (see slides 39 and 40) in the above link (I used storm sewer installation date).
So what can we expect when we look at the flood risk profile across Canadian cities based on these Servicing Eras? First, we can expect to see a vast variation from city to city based on its growth and servicing history. Using Statistics Canada data on from the 2016 census for Ontario, we can use the date of housing construction as a surrogate for the date of municipal servicing - this approach has limitations because housing may be in place before servicing in isolated cases (i.e., servicing is newer than the housing), and servicing may be upgraded over time (i.e., capacity upgrades to original servicing). The graph below looks at housing/construction eras for census metropolitan areas (CMAs) or smaller geographic units.
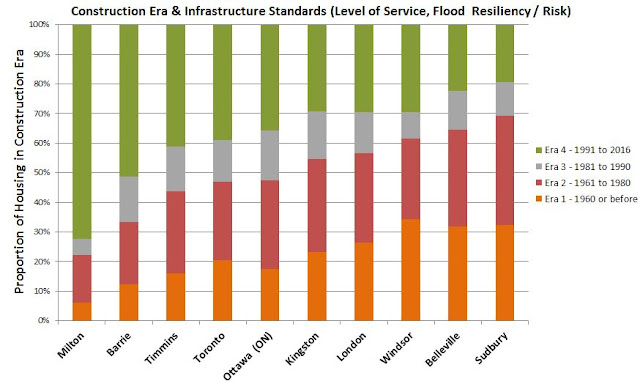
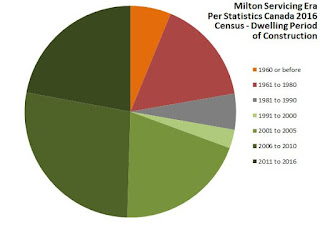
We see that areas with more growth (e.g., Milton, Barrie) have less than half the proportion of high and highest risk housing stock (Eras 1 and 2) compared to other low growth areas (e.g., Sudbury, Belleville). The Milton breakdown is shown on the left. Milton has over 70% Era 4 resilient housing built and serviced after 1991 (green shaded slices of the pie), whereas Sudbury has less than 20% of housing in the group. The Sudbury breakdown is also shown on the left.
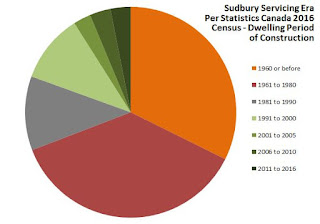
Milton shows significant growth in the 2001-2005, 2006-2010 and 2011-2016 periods once water and wastewater servicing was available to this part of the Halton Region (previously well water supply limited growth). In contrast, Sudbury shows limited growth post 2006, reflecting perhaps a slowdown in the resources/mining sector following the 2008-2009 recession.
The bar chart totals were for some entire census areas encompassing several municipalities (e.g., the Toronto CMA includes may municipalities including in York Region, Peel Region and Halton Region). Within the CMA or municipalities themselves, the infrastructure construction era will also vary (see Toronto watermain installation date in the slide noted above). For example in the Toronto CMA 47 % of housing is within Era 1 and 2, while in the City of Toronto itself 64 % of housing is within those eras, reflecting older housing stock and servicing in Toronto compared to newer communities such as Mississauga, Markham, Vaughan, etc.. Toronto also has less resilient Era 4 housing stock compared to its CMA, i.e., 26 % vs 39 %, respectively.
The type of housing must be considered where there is a high proportion of condominium / apartment dwelling types that do not have basements with the same single family dwelling back-up risks and that typically have no riparian flood risks. The City of Toronto housing units are 39% apartment, including many new condominium units, while in the broader Toronto CMA, apartments account for 29%. As a result, the resiliency of areas with a high proportion of newer apartments may be slightly overestimated.
What's next - mapping the Servicing Era and flood risk profile at a census tract level perhaps and netting out the effect of apartments and then adding in other local risk factors that are readily available.
****
And here we go with some neighbourhood variability in era of construction to show the variability in land use planning, subdivision infrastructure servicing and dwelling construction practices across several regions in Canada. The average neighbourhood age is based on Statistics Canada data at a census tract scale - sometimes that is too small a scale to assess risk and sometimes it too big, and sometimes it does not align with infrastructure system servicing boundaries. So what I'm saying is its a high level general characterization of resiliency, and one would have to drill down into specific infrastructure systems to see street by street resiliency :
Vancouver:
Calgary:
Golden Horseshoe / Toronto / Hamilton:
Ottawa / Gatineau:

Halifax:
Other factors that characterize flood risk can also be considered, including overland flow and topographic slopes that contribute to direct surface flooding and also inflows to sanitary sewer systems (e..g, via reverse slope driveways and low opening, or windows and lower level walkouts that ultimately drain to floor drains and the sanitary/wastewater collection system).
The following images show the estimated 100-year overland flow spread for drainage areas up to 10 hectares in Toronto. Catchments with low slopes are also shown as these have been shown to influence the maximum flood density reported following extreme rainfall events.
The first map shows the Newtonbrook area of North York where the overland conveyance limitations are clearly apparent. It would appear that the topographic drainage limitations (low slopes, no overland outlet) combined with the age of construction (with partially-separated sanitary sewers) results in the high relative flood risk.
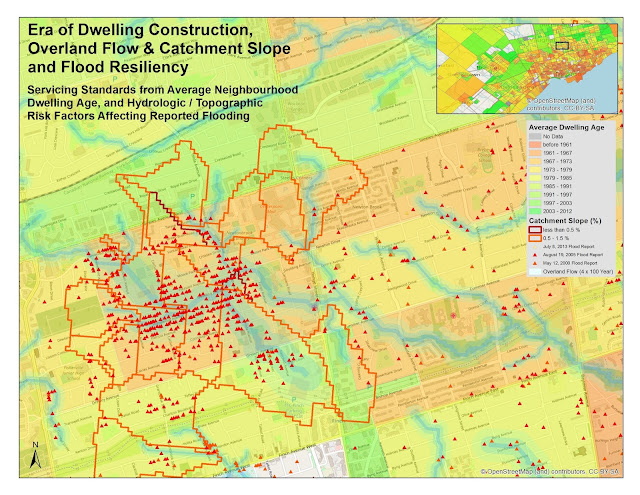
I have to say it is tempting to cherry pick the map area to prove my hypothesis is correct in terms of flood risk factors. The west end Toronto map shows the limitations in flood risk factors. For example the greatest flooding in May 2000 was not in the low slope catchments or the oldest construction area but rather in a cluster north west of Eglinton Ave West and Islington Avenue. The oldest area to the south shows one flood cluster on the overland flow path but not throughout the old area for the May 2000 event. So is construction age alone a good indicator of risk? Overall it is (analysis of all Toronto flood reports proves it is relevant), but it breaks down at the neighbourhood or sub-neighbourhood level as a predictor of risk. The best indicator of risk? Past flooding. Why? Because the complex reality of hydrologic and hydraulic systems and building construction cannot be readily simplified into list of risk factors - there is too much variability, too many exceptions and too much interaction between known and unknown factors to identify risk at a fine spatial scale.
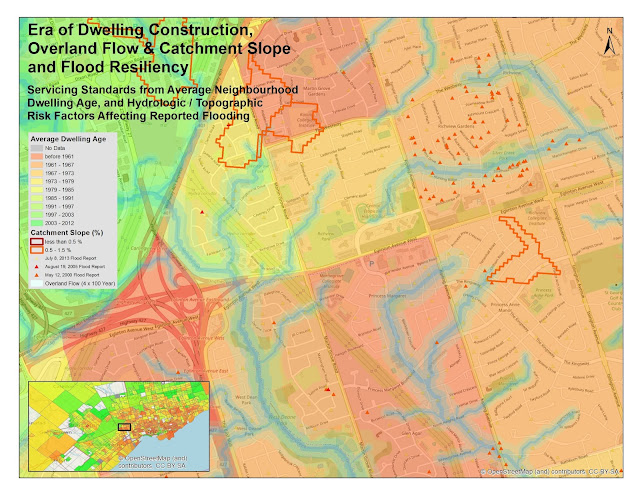
The final map below shows another west end area where 10 hectare overland flow paths help explain some flood clusters but not others. Slope does not seen to be a driving factor where the overland drainage area is small (near Trethewey Drive), but may be a more significant factor on Jane Street where the overland flow area is more significant. What this shows is that it is combinations of factors that accentuate overall flood risk. If one factor is quite severe, it can trigger a flood cluster like east of Dufferin Street, north of Lawrence Avenue West where the age of construction is newer, slopes are good but the overland flow system behaviour alone is enough to trigger risks. Like there previous map, this one shows cluster that are off the major overland flow paths - so these do characterize risk on an aggregate basis overall, but not always at a local spatial scale.
This is perhaps the best example of overland flow risks coming to life like a 'giant tiger' during a severe storm. The picture is from Twitter at .. ummm .. the Giant Tiger store on Kipling Avenue. The store is immediately on the overland flow path - not a huge drainage area, but the building effectively blocks the flow path. The low slopes (orange polygon) around the store suggest it is in a 'bowl' (like Newtonbrook in the image above), which means the overland flow has 'nowhere to go' - good overland slopes help 'move' runoff during an extreme event.
Looking at Mississauga, and reported flooding from July 8, 2013 one can see higher concentrations in older areas closer to Lake Ontario and in Malton (top of inset map). Newer areas that appear to have low densities of flood reports may in fact just be commercial properties with no basements and with owners or tenants who did not report flooding to the City after the storm event (e.g., areas surrounding Pearson Airport). It does appear that relatively less flooding was experienced in newer subdivisions to the west (e.g., Erin Mills).

Adding other factors such as overland flow paths to the Mississauga flooding and construction era maps shows again, like in some Toronto maps, that the combination of factors drives flood risks. Clearly higher densities of flooding within older neighbourhoods (census tracts) can be found along overland flow paths (note regulated floodplains are not shown). The tributary west of Cawthra Road between the QEW and the CNR in the Mineaola neighbourhood shows a clear line of reported flooding along the overland flow path. Other local areas in the Mineola neighbourhood do not have a high degree of reported flooding, despite the older age of building construction and servicing standards - some flooding in that neighbourhood is associated with Cooksville Creek riparian flooding risks.
Edmonton has mapped overland flooding risk areas to help educate residents on flood risks. The map below illustrates the variability in dwelling construction date across census tracts.
The following map shows areas with surcharged sewers and surface ponding risks based on the interactive map available here:
http://edmontonjournal.com/news/local-news/first-defence-new-epcor-ranking-scheme-may-wake-up-neighbourhoods-to-flood-risk.
What does it show? Old areas south of the rail tracks have higher sewer surcharge risk (red pipes), corresponding to the old 'grid pattern' development. Newer areas to the north, especially north of 153 Avenue NW have very few sewer surcharge risk (i.e., fully separated sanitary sewer servicing) - those are the areas with the modern 'wiggly' road patterns. Its all coming together !
The City of Windsor experienced extensive flooding in both 2016 and 2017. The following map illustrates August 2017 flood reports.
The era of construction is shown in the map below. It would appear that oldest areas had the highest flooding reports, and newest areas (e.g., in the west) had lower flood density.
Looking back at Mississauga, we have analyzed the flood density in residential development areas by era of construction, using weighted average construction date in each census tract. The flooding locations were estimated through digitizing and therefore likely underestimate reports in the highest density areas due to overlapping symbols. Nonetheless, a strong trends appears in the data with a lower density of flooding for 1980-1989 construction compared to pre-1980 construction - this reflects the benefits of full sanitary sewer separation and more advance master drainage planning. Post-1990 construction areas show even lower flood density that 1980-1990. The ratio of flooding density in the three eras of pre-1980, 1980-1989, 1990-present was 3.1 - 1.8 - 1. Unlike the Markham densities above that are based on total dwelling counts, the Mississauga densities area are-based and therefore if modern dwelling densities are higher, the relative flooding would be even lower for more modern construction (i.e., more dwelling per area, resulting in even lower flooding per dwelling). The following map illustrate the residential areas (with the exception of Malton), colour-coded by construction era and estimated flood locations.

Looking a little closer at Mississauga and the major overland drainage system, con can see the influence on reported flooding. Older construction areas do not have uniform flood risks - while risks are higher overall, on an aggregate basis in older vs modern drainage systems, with the older areas there are distinct clusters of flooding. Often those clusters are along a regulated valley feature or along an major overland flow path upstream of the regulated area - several examples can be seen in the map. The inset at the top right is the Malton area of Mississauga - flooding clusters are apparent along the major overland flow path beyond regulated areas - this does not necessarily mean flooding was overland, pluvial flooding, but that the major system conveyance limitations stressed the sanitary sewer system, for example, with extraneous inflows in an area that already has high infiltration flows.
***
Two key flood risk factors are combined in the following map showing the City of Waterloo. Spatial analysis of overland flow path hydrologic characteristics are intersected with city sanitary sewer system age and inferred infiltration and inflow risk to identify risk areas of interest - these can be assessed through further study, whether that be investigation of critical system conditions (e.g., CCTV inspection), monitoring of flow stresses to confirm inflow potential sewer surcharge risk magnitude, to modelling / quantification of flood risks, or further risk characterization through investigation of past flood claims and reports. Average dwelling age of construction in census areas is labelled to show the general correlation of broad neighbourhood risk factors (i.e., construction era is a surrogate for sewer and overland drainage design standard resiliency). Individual dwellings near the overland flow path are shown in red, indicating specific local risks within new and old subdivisions.
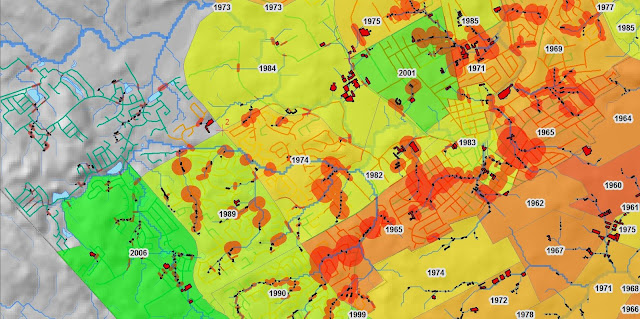 |
City of Waterloo - Example Urban Flood Risk Factor Review Considering 100-Year Overland Flow Risks and Sanitary Sewer Resiliency Based on Construction Era and Inflow / Infiltration Potential (Excludes Riverine Flood Risk and Properties in Regulatory Flood Plain) |
Methods for assessing urban and riverine flood risks from "Flood Plains to Floor Drains" are discussed in
a previous post.